Table of Contents
Page created on March 7, 2018. Last updated on December 18, 2024 at 16:55
Summary
- Brain and nerve cells use 60-70% of their energy for transport processes, mostly for the Na+/K+ ATPase, to uphold the action potential
- The brain uses almost exclusively glucose as an energy source, but can use ketone bodies as well
- PET (positron emissions tomography) can be used to detect large amounts of glucose in the body. Glucose normally accumulates in tumours, among other places.
- Muscles use mostly (>85%) fatty acids as an ATP energy source during rest, but glycogen, glucose and creatine phosphate during hard work.
- Anaerobic (or ischaemic) conditions in muscle cells turns off oxidative phosphorylation, β-oxidation and TCA.
- Triacylglycerols cannot cross the adipocyte cell membrane. They must be degraded in the cell for export as fatty acids.
- The synthesis of triacylglycerols in adipocytes requires glucose (to create glycerol)
- Triacylglycerols compose by far the largest energy reserve in the body
Brain and nerve cells
Nerve cells don’t really divide. They mainly require energy for upholding the action potential, by transporting ions in and out of the cell, against the electrochemical forces. The sodium-potassium ATPase (Na+/K+-ATPase) uses most of this energy. Neurotransmitter biosynthesis also requires some energy.
The brain uses mostly glucose for energy, because the blood-brain-barrier limits what can be transported in and out of the brain. This barrier has a specific transporter for glucose, but not for fatty acids. The brain can use ketone bodies in case of long-term hunger or untreated diabetes.
Positron emissions tomography
PET can be used to see where in the body glucose is accumulated. The patient is fed a solution with a special type of glucose the oxygen atom on carbon number 2 is substituted with a special isotope of fluoride, F18. This glucose is called 2-F-2-deoxyglucose, or FDG for short. FDG is so similar to glucose that it is transported into the cell by glucose transporter. It is then converted by hexokinase into 2-F-2-deoxyglucose 6-phosphate by hexokinase. However, it cannot be converted into fluoride-fructose, because this reaction requires an oxygen in place of the fluoride, so it doesn’t proceed into glycolysis.
FDG is toxic in high doses. However, the fluoride isotope is radioactive and will eventually lose a positron by positron emission. This will convert F18 into oxygen (which is normally supposed to be there), so the molecule is no longer toxic, and can proceed into the glycolysis. The positron emission can be detected by sensors, which creates the resulting 3D image.
Muscle cells
Muscle cells need ATP to release the actin-myosin binding. These cells primarily use glucose, fatty acids and ketone bodies, but not lactate as previously believed. During rest, muscles use primarily (more than 85%) fatty acids for energy. Fatty acids give energy slowly, so they are mainly used during rest. During hard muscle work, the process of degrading fatty acids is too slow. That’s why, during active use, muscle cells use sources that give energy quickly, like creatine phosphate and glycogen.
During hard muscle work, the muscle cells don’t have time to receive the oxygen it needs, so they will work in hypoxia. The respiratory chain, β-oxidation and citric acid cycle all require oxygen, so in these conditions, the cell will turn to glycolysis for ATP production. The energy will come from substrate-level phosphorylation, muscle glycogen, blood glucose and the Cori’s cycle.
Adipose tissue
Adipose tissue stores most of the energy in the body, in the form of triacylglycerols. However, these molecules are very large, and therefore cannot leave the cell in their current form. They have to be degraded inside the cell, to fatty acids and glycerol, so the free fatty acids can leave the cell and enter the bloodstream.
The synthesis of triacylglycerol requires glucose. This glucose is used to synthesize glycerol, the backbone of triacylglycerols. However, the adipocytes need glucose for fat degradation as well. The intracellular glucose level is essential for influencing whether the adipocyte releases fatty acids into the blood or not. In the case of untreated diabetics, which produce no or ineffective insulin, the adipocytes never take up glucose, because the GLUT4 transporter is activated by insulin. Without glucose in the cell, the adipocytes cannot break down fat.
Blood
The main cell type in blood is the erythrocyte, which contain no mitochondria. Erythrocytes are therefore entirely dependent on substrate-level phosphorylation (glycolysis).
The normal range of blood glucose is 60-90 mg/100mL.
How can a hormone have different effects on the same metabolic pathway in two different tissues?
Take epinephrine for example. It activates glycolysis in the cardiac muscle but deactivates it in the liver. What is the mechanism behind this? It’s normally due to isozymes, enzymes that catalyse the same reaction but are different in other ways.
In epinephrine’s case, PFK-2/F26BPase isozymes are involved. Recall that PFK-2 and F26BPase are not actually separate enzymes, but rather two enzymatic activities on the same protein. This protein is slightly different in liver and in cardiac muscle. See the drawings below. In the liver, the protein has a serine residue near the N-terminus, where the PFK-2 activity lies. This serine residue has a hydroxyl group that can be phosphorylated, which will inhibit the PFK-2 activity.
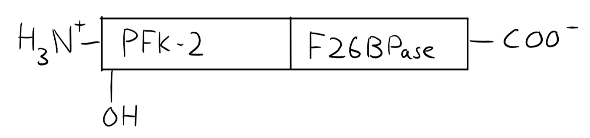
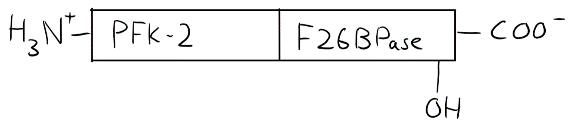
In the cardiac muscle, the PFK-2/F26BPase protein is slightly different. The hydroxyl group that can be phosphorylated is now close to the C-terminus. Phosphorylation will now inhibit F26BPase activity.
Now, if epinephrine is active, both the liver cell and cardiac muscle cell will have their Protein Kinase A’s activated, which will phosphorylate PFK-2/F26BPase in both tissues. However, the phosphorylation will happen at different places on the protein, which will have different effects on it. In liver, PFK-2 will be inhibited, so F26BP level in the cell will drop, which inhibits glycolysis. In cardiac muscle, F26BPase will be inhibited, so F26BP level in the cell increases, which activates glycolysis.
Note that the PFK-2/F26BPase protein in skeletal muscle is not regulated by phosphorylation! This protein in the skeletal muscle has no serine residues to be phosphorylated. Instead, epinephrine activates glycolysis in the skeletal muscle through a different pathway:
Epinephrine activates adenylyl cyclase, which activates PKA. PKA then activates glycogen phosphorylase kinase, which again activates glycogen phosphorylase. This enzyme will degrade glycogen into glucose 1-phosphate. Phoshoglucomutase will then convert glucose 1-phosphate into glucose 6-phosphate, which allosterically activates the glycolysis.